Applications of Intravascular Lithotripsy in the Management of Peripheral Artery Disease: A Review of the Literature
Syed Hamza Mufarrih, MD1; Rayaan A. Yunus, BA2; Mahnoor Sohail, MBBS3; Mohammad Saud Khan, MD4; Nada Qaisar Qureshi, MBBS1; Arti Saraswat, MD, MPH5; Glenn A. Hirsch, MD, MHS6; Srini Tummala, MD7; Mazen S. Albaghdadi, MD, MSc8; Avnish Tripathi, MD, PhD, MPH9
ISSN: 2694-3026
J CRIT LIMB ISCHEM 2024:4(2):E34-E43. doi:10.25270/jcli/CLIG23-00018
Abstract
Peripheral artery disease (PAD) is a common atherosclerotic disease associated with significant morbidity. The definitive treatment for advanced chronic limb-threatening ischemia typically includes endovascular revascularization strategies. However, vascular calcification without optimal vessel preparation prevents optimal lumen gain with balloon angioplasty and, therefore, optimal stent expansion. In addition, the efficacy of drug-eluting technology is significantly reduced due to the inability to diffuse through the calcification into the media. Intravascular lithotripsy (IVL), an emerging technology using acoustic shock wave energy, is an adjunct to transitional endovascular revascularization techniques for heavily calcified PAD. In this manuscript, the authors have reviewed the role of IVL as an adjunct to endovascular revascularization in treating PAD.
J CRIT LIMB ISCHEM 2024:4(2):E34-E43. doi:10.25270/jcli/CLIG23-00018
Key words: intravascular lithotripsy, peripheral artery disease
Peripheral artery disease (PAD) arises from the progressive development of occlusive atherosclerosis, predominantly in the lower limb peripheral vasculature.1 PAD has been increasingly recognized as an important cause of cardiovascular morbidity and mortality. Over the past decade, there has been a significant rise in hospital admissions for PAD globally, with more than 230 million admissions recorded in 2015, mainly due to an aging population and the increase in diabetes and chronic kidney disease.1 The estimated prevalence of PAD in the United States is approximately 7%, affecting 8.5 million adults.1
Intermittent claudication is the most common presentation of peripheral arterial occlusive disease; however, an estimated 50% to 90% of patients with definite intermittent claudication failed to report the symptom to the clinician because a decrease in walking distance, and atypical symptoms such as leg cramping and pain, are assumed to be a normal part of aging.2-4 When left untreated, PAD-associated atheromatous plaques continue to cause inflammation within the vessel wall with subsequent plaque calcification, causing subacute obstruction, progressive symptoms, and chronic limb ischemia.5 The disease progression may be complicated by plaque rupture with acute subtotal or total vessel occlusion presenting as acute limb-threatening ischemia.5
Initial treatment approaches for PAD typically involve lifestyle modifications, exercise therapy, and guideline-directed medical therapy aimed at modifying risk factors such as lipid levels, glycemic control, and blood pressure. Endovascular and surgical interventions should be considered as definitive therapies for patients who do not respond to conservative treatment and continue to experience lifestyle-limiting claudication symptoms or develop chronic limb-threatening ischemia (CLTI).5
Endovascular revascularization has gained widespread acceptance as a primary treatment strategy for select patients with PAD. However, the presence of vascular calcification often leads to suboptimal outcomes. For example, intimal and medial calcification can impede lumen expansion during balloon angioplasty and restrict stent expansion. Moreover, calcification poses challenges in achieving optimal drug delivery with drug-eluting technologies such as drug-eluting stents (DES) and drug-eluting balloons (DCB) 6 As a result, effective vessel preparation techniques are necessary to address arterial wall calcification through debulking or fracture interventions.
Intravascular lithotripsy (IVL) has emerged as a vessel preparation device for heavily calcified arteries during endovascular therapies.8 IVL utilizes multiple emitters mounted on a traditional angioplasty balloon catheter to deliver pulsatile acoustic pressure energy. This approach facilitates the fracture of superficial and deep calcium without compromising local soft tissues and minimizes the risk of emboli liberation.9 In this manuscript, we provide a comprehensive review of the role of IVL as an adjunct to endovascular revascularization in the treatment of PAD.
Historical Perspective of Lithotripsy
The concept of lithotripsy was first introduced by Bavarian surgeon Franz von Paula Gruithuisen in 1813, leading to the invention of the lithotrite, a surgical instrument used to crush kidney stones inside the bladder, by Jean Civiale in 1832.10 The first successful lithotripsy procedure took place in 1834 at the French Academy in Paris.10 Although lithotripsy briefly gained popularity as an alternative to lithotomy, the introduction of general anesthesia shortly after by William Morton in 1846 shifted favor back to lithotomy until the late 1980s.10
In 1971, Haeusler et al. demonstrated the use of shock waves, strong ultrasonic pulses propagated in a water cylinder, to destroy kidney stones.10 In 1975, Claude Dornier applied this technique extracorporeally and successfully fragmented kidney stones in vivo in experimental animal models.10 Walter Brendel, in 1980, performed the first successful human extracorporeal shock wave lithotripsy at the Institute for Surgical Research in Munich.10
The idea of using lithotripsy intravascularly to target calcified intracoronary and peripheral arterial plaques was first proposed by Daniel Hawkins in 2007.11 The integration of both high- and dual-frequency technology, the development of laser-assisted IVL devices, improved ergonomics, image guidance, and versatility laid the foundation for demonstrating the safety and efficacy of this technique in several clinical trials.11 The use of IVL devices to treat intravascular calcification was officially approved by the FDA in 2016 (Figure 1).11
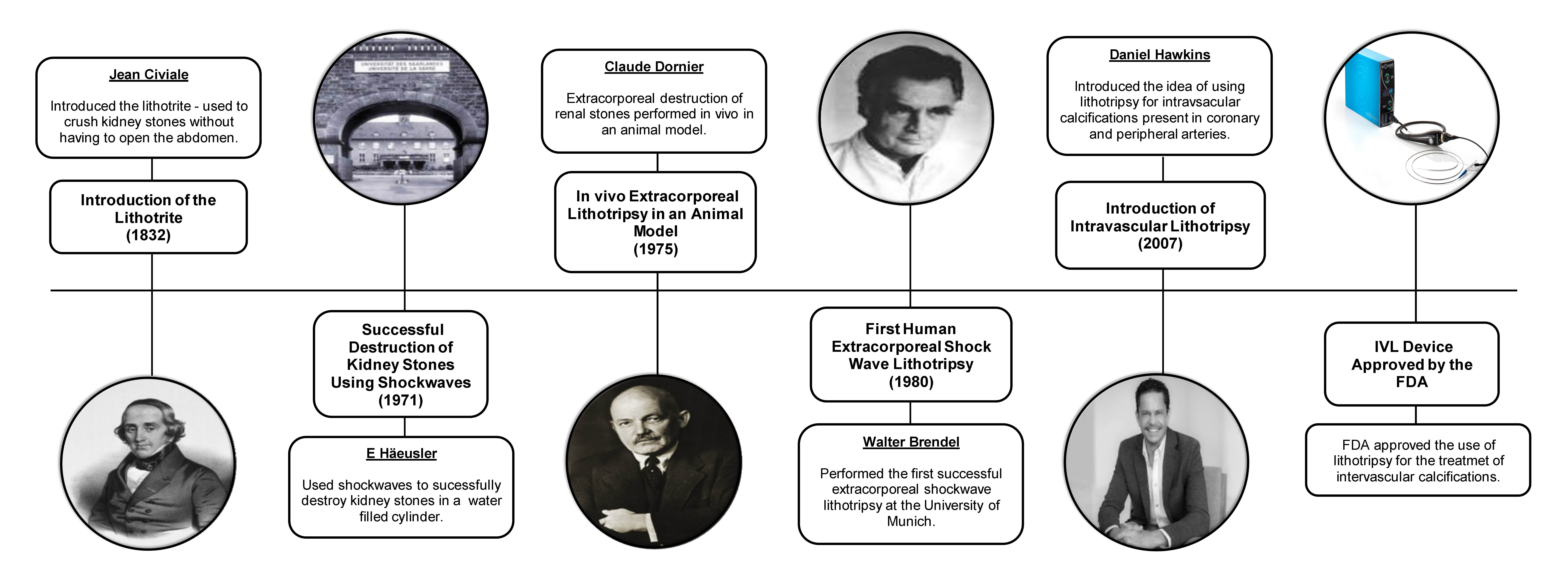
Principles of IVL in Peripheral Artery Intervention
The IVL balloon, which includes lithotripsy emitters, is mounted at the tip of the peripheral IVL balloon-catheter system and serves as a pressure wave emitter that converts electrical energy from the external pulse generator into sonic pressure waves.9 These transient acoustic circumferential pressure pulses selectively fracture calcium within the vascular plaque, altering vessel compliance.9 The shock waves are characterized by a short duration of 5 µs, with positive and negative pressure peaks and troughs components.9 To perform the procedure, the balloon catheter is advanced to the target lesion after establishing vascular access. The balloon is then inflated at low pressure (4 atm) to avoid barotrauma, and high-energy pulses are delivered. Following this, further balloon dilation (6 atm) is performed before deflating the balloon to allow for resumption of distal perfusion.12 This process can be repeated for a maximum of 10 cycles per balloon, totaling 300 pulses per catheter.12 Post-dilatation is an option after IVL, ensuring adequate lesion preparation and dilatability of the target lesion, before stent implantation or other modalities such as DCB angioplasty.12 For detailed preparation and procedural steps, refer to Table 1; typical indications for IVL are described in Table 2 and Figure 2.
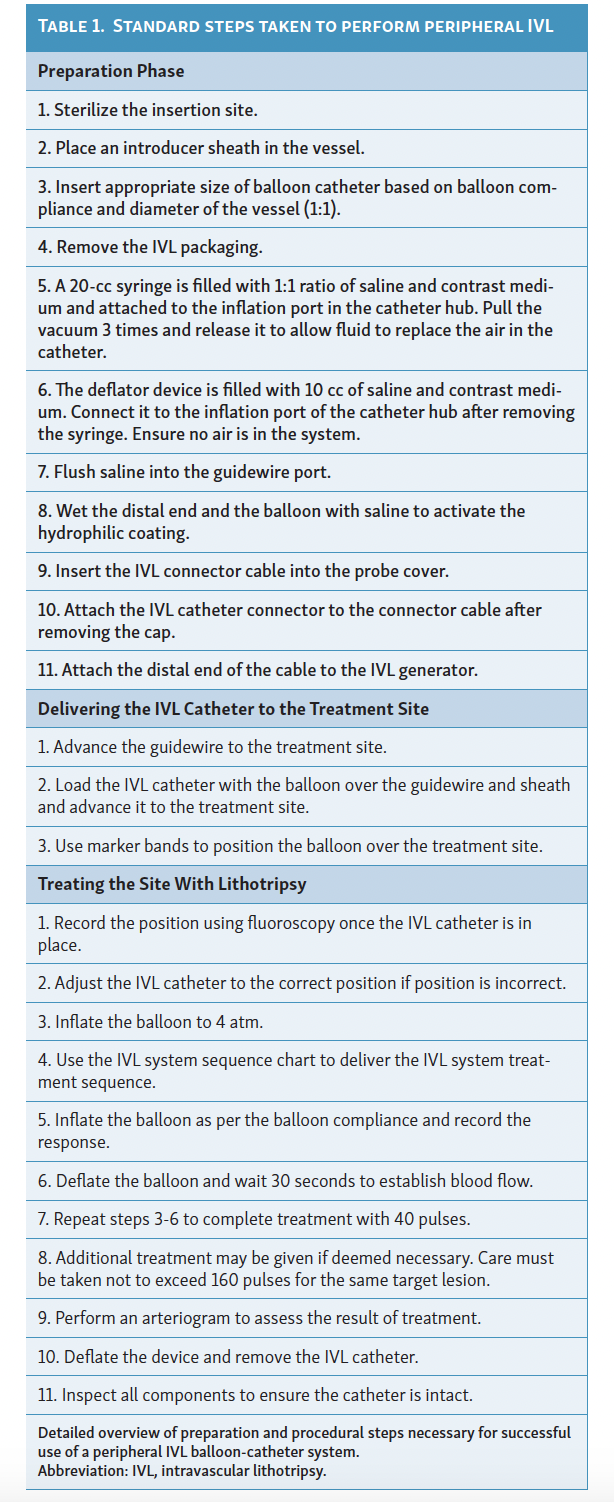
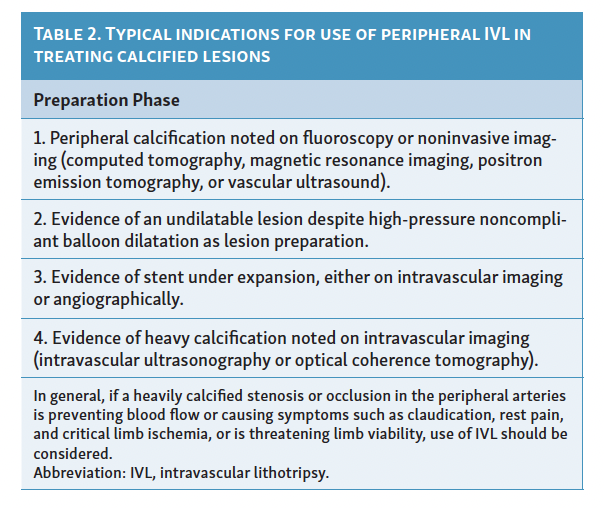
At the time of initial FDA approval, the peripheral IVL balloon catheter consisted of an over-the-wire balloon ranging from 3.5 mm to 7.0 mm in diameter (0.5 mm increments) and 60 mm in length, requiring a 6F or 7F sheath depending on the balloon diameter.12 The newest catheter, M5* (Shockwave Medical), is available with a longer length (135 cm) and goes up to 8.0 mm (Table 3) (Figure 3).12 The invention of smaller IVL balloon catheters, particularly the M5+ catheter, with a hydrophilic coating, lower crossing profiles, and a 135 cm shaft length, has enabled the treatment of contralateral distal peripheral calcified lesions.12 The refined design of the M5+ catheter has also optimized the location of energy emission, resulting in increased impact on calcified plaques by altering the location of the middle two lithotripters to focus shock wave energy in the middle of the target field.12 Advancements in catheter technology have led to FDA approval for IVL treatment in various peripheral vascular beds, including the femoral, iliac, popliteal, infrapopliteal, celiac, mesenteric, and renal arteries.12
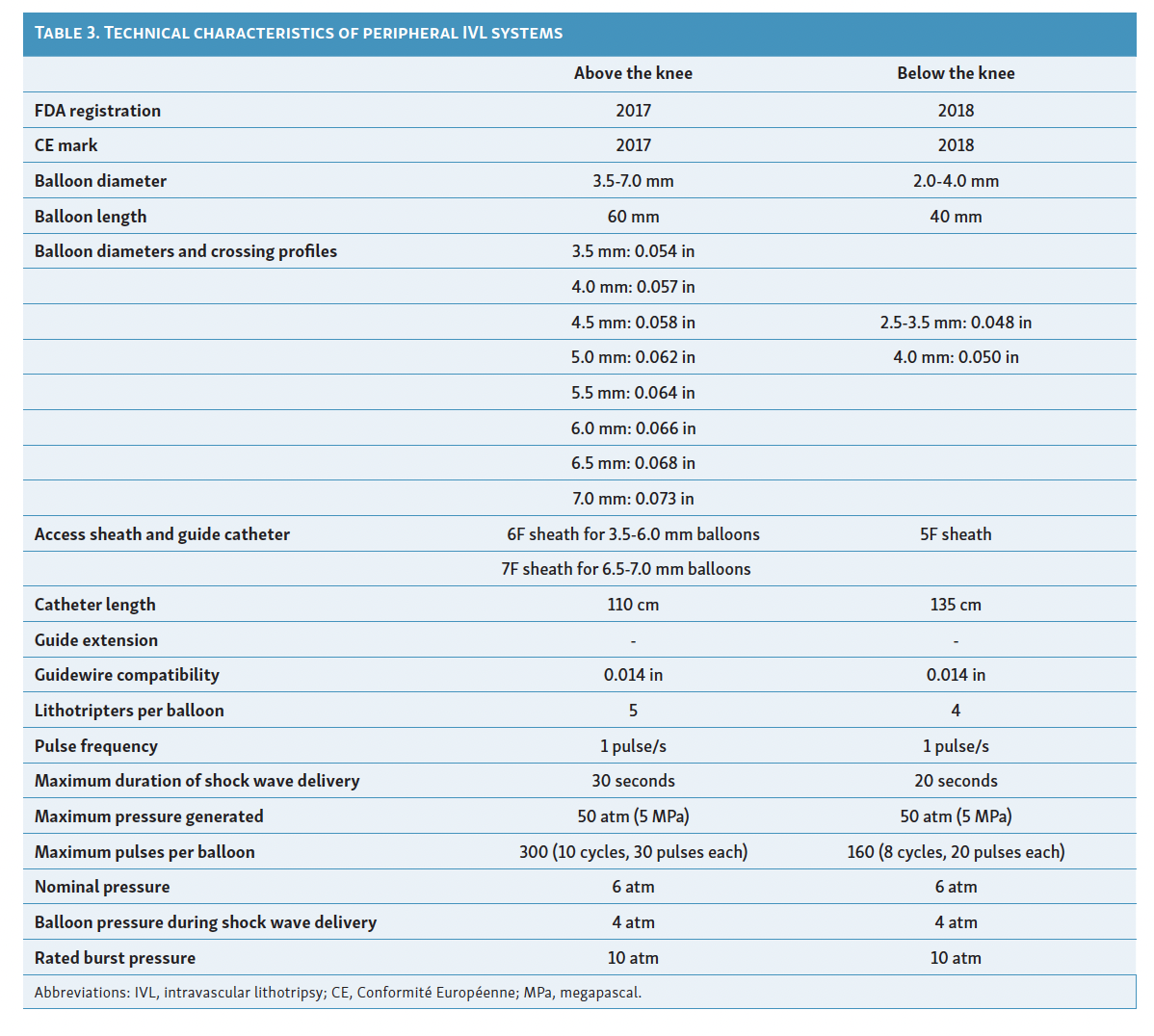
When performed with the proper preparation and without contraindications (unable to pass guidewire across the lesion, treatment for in-stent restenosis, or for use in carotid, or cerebrovascular arteries), IVL has proven to be a safe and effective form of therapy (Table 2). Several mechanisms may contribute to the fragmentation of calcific plaque by IVL, including the following:
1. Compressive circumferential forces generated by variable propagation of the acoustic shock waves causes axial splitting in the solid calcified plaque and the surrounding soft tissue.13
2. Collapse of cavitation bubbles within the saline-contrast-filled balloon that impacts the surface of the calcific plaque.13
3. Progressive expansion of microfractures into macrofractures due to the cumulative impact of repetitive shock wave pulses causes fatigue.13 Furthermore, fracturing calcium may augment delivery of antiproliferative agents from DCB or DES to the vessel wall.
While shock wave energy may affect the surrounding vascular tissue and potentially cause inflammation and injury, a recent in vivo study on iliac and femoral arteries showed no difference in inflammation or soft tissue damage between balloon angioplasty and IVL, despite using 180 energy pulses, which is more than the recommended amount.14 Additionally, as IVL does not involve displacing plaques, the risk of embolization is lower compared with atherectomy devices such as the SilverHawk and TurboHawk plaque excision systems (Medtronic).9
Clinical Evidence for IVL in PAD
Since its introduction as a treatment option for PAD in 2016, IVL has limited available evidence to support its efficacy. Although initial results show promise, there are few comparative studies with traditional endovascular therapies and more are needed for a clearer definition regarding the effectiveness of IVL in heavily calcified vessels. Among the currently available evidence, only 4 studies have attempted to classify vascular calcification using the Peripheral Academic Research Consortium (PARC) criteria in the context of lithotripsy device utilization. Table 4 and Table 5 summarize the characteristics and results of these studies assessing the use of IVL in patients with PAD. 15-25
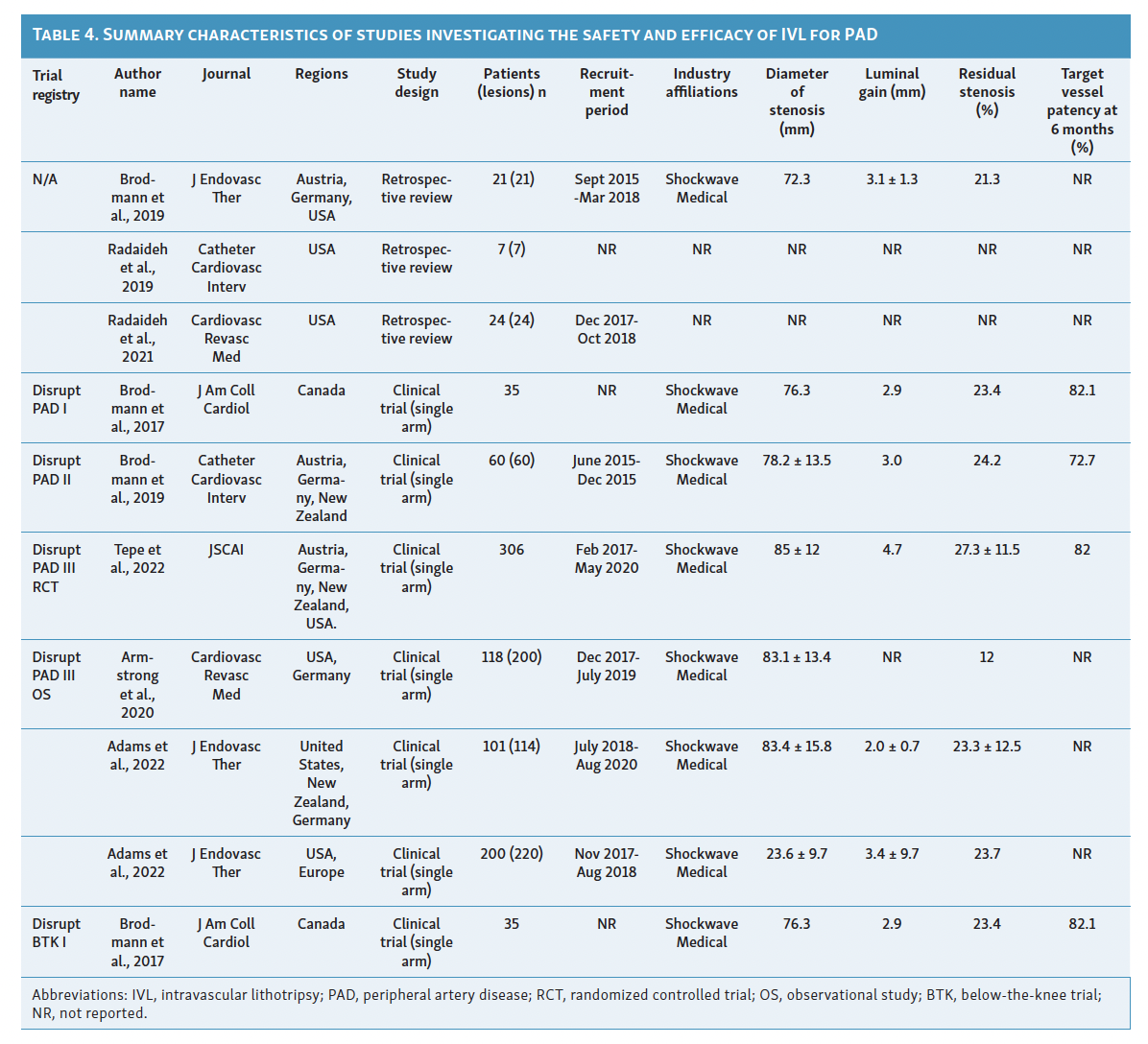
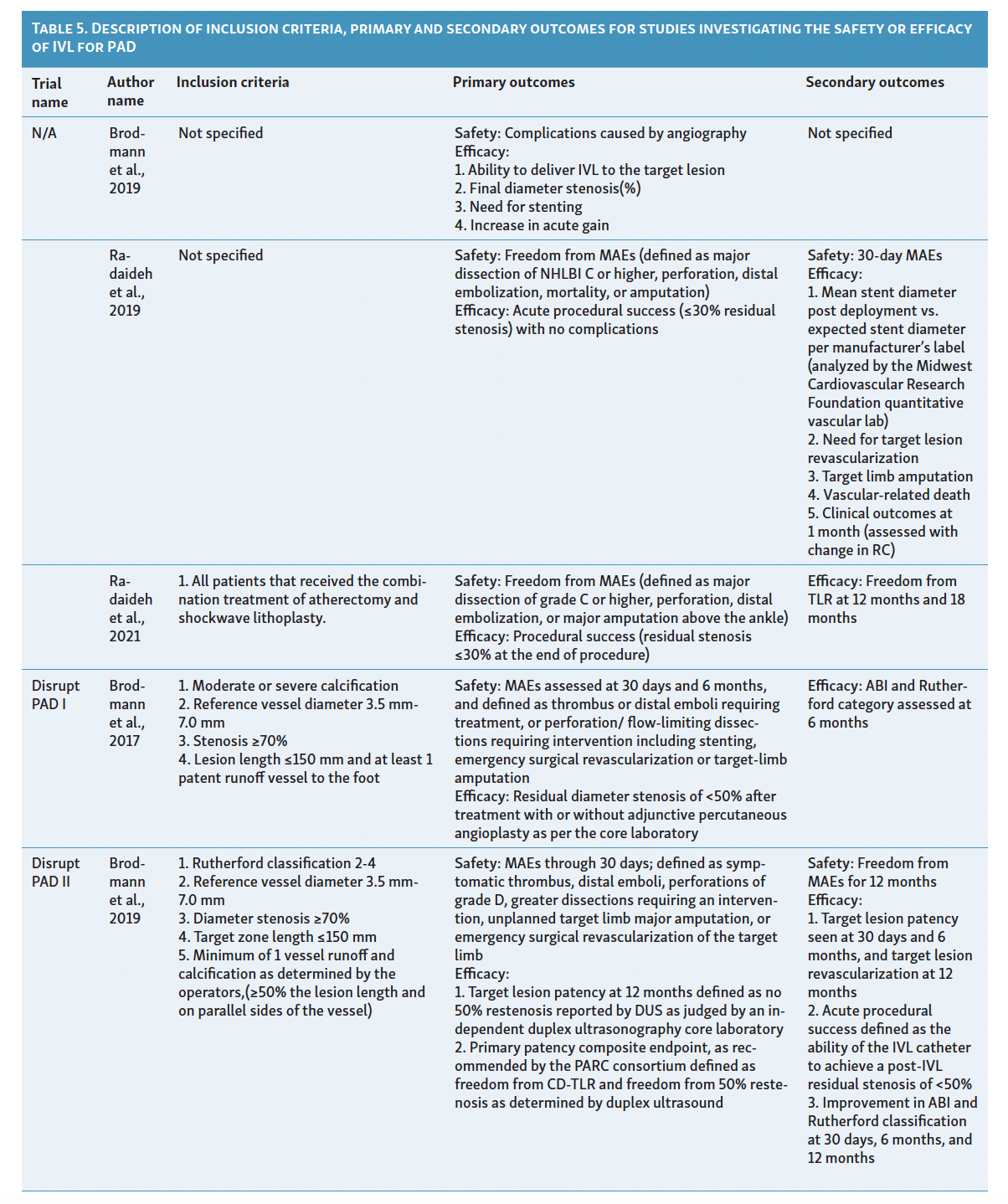
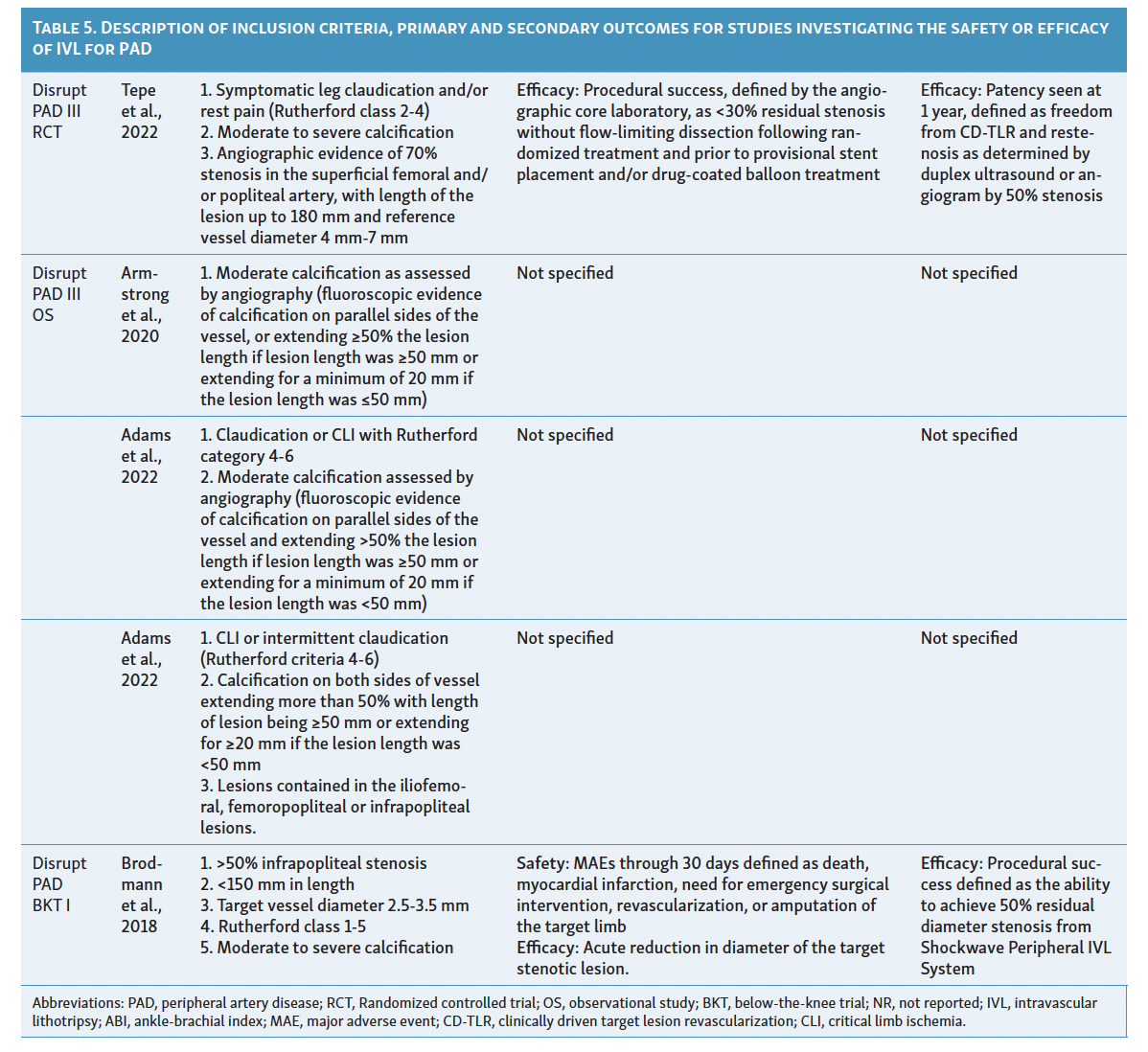
IVL in Femoropopliteal Arterial Disease
The safety, performance, and efficacy of IVL in treating femoropopliteal artery disease have been assessed in the Disrupt PAD trials I, II, and III.15-17 These trials, sponsored by Shockwave Medical, were multicenter, prospective studies (PAD I and II were nonrandomized, PAD III randomized) that included patients with angiographic evidence of 70% or higher stenosis, de novo superficial femoropopliteal artery or popliteal artery target lesion, fluoroscopic evidence of calcification more than 50% of lesion length, or Rutherford classification 2 to 4.15-17 These trials excluded patients with planned target limb major amputation, ischemic ulcerations, renal disease, in-stent restenosis within 10 mm of the target zone, significant stenosis (>50%) or occlusion of inflow tract before the target zone is not successfully treated, and premarket, single gangrene.15-17
The Disrupt PAD I trial, a premarket single-arm prospective trial initially presented in 2017, demonstrated 100% procedural success in calcified stenotic target vessels with lesion lengths of 150 mm.15 Safety outcomes of interest included surgical revascularization, amputation, distal embolization, or interventions for perforation/flow-limiting dissections requiring stenting.15 Among the 35 participants in this trial, only 23.4% residual stenosis was observed, with sustained improvement in Rutherford category scores at the 6-month follow-up and no major adverse events.15
Following Disrupt PAD I, Disrupt PAD II became the first postmarket, prospective, multicenter, nonrandomized study to directly evaluate the safety and effectiveness of IVL therapy in a larger cohort over a longer duration (1-year follow-up).16 The trial involved 60 participants with 78% vessel stenosis and a mean vessel length of 98 mm.16 Similar to Disrupt PAD I, this study demonstrated 79% target lesion patency at 1-year follow-up, with no postprocedural perforations, abrupt closure, or slow flow/no-reflow reported, except for 1 case of grade D wire-induced dissection treated with stent placement.16 Notably, experts observed improved target lesion patency when using an IVL balloon size to artery size ratio of 1.1:1 and recommended at least 1 cm balloon overlap if multiple inflations are required due to longer lesions to prevent a geographic miss.16 These technical improvements were rapidly adopted in global guidelines.12
The Disrupt PAD III trial, a single-blinded, double-armed global randomized controlled trial, compared IVL with percutaneous transluminal angioplasty (PTA) in severely calcified femoropopliteal lesions.17 The trial demonstrated significantly greater primary patency at 1-year (80.5% vs 68.0%, P=.017) and 2-year (70.3% vs 51.3%, P=.003) follow-up in the IVL arm.17 Patients in the IVL group also required less provisional stenting (4.6% vs 18.3%, P<.001) and showed similar rates of freedom from target lesion revascularization (95.7% vs 98.3%, P=.94) compared with the PTA group.17 This trial provided the first evidence of IVL’s efficacy against PTA in treating moderate-to-severe PAD.17 Additionally, the Disrupt PAD III observational registry has evaluated the safety and efficacy of IVL in combination with other endovascular therapies. A report by Radaideh et al demonstrated the safety and effectiveness of combined rotational atherectomy and IVL treatment, resulting in a final minimal luminal diameter of 4.7 ± 0.8 and an acute gain of 2.5 mm.18 Similar findings were observed when IVL was used in conjunction with other balloon-based technologies.19
IVL in Infrapopliteal Arterial Disease
Treating below-the-knee (BTK) or infrapopliteal artery disease is often challenging due to significant elastic recoil and the presence of diffuse calcification. The Disrupt BTK I trial, the first global, multicenter, prospective, nonrandomized trial, showcased the safety and feasibility of IVL in treating calcified infrapopliteal stenosis using the M5 peripheral IVL catheter (Shockwave Medical).20 The eligibility criteria and outcomes of interest aligned with the Disrupt PAD trials.15-17, 20 This trial demonstrated a remarkable 46% reduction in the diameter of target lesions, resulting in a mean residual stenosis diameter of 26.2% and a luminal gain of 1.5 ± 0.5 mm.20 Vascular complications were minimal, with only 1 occurrence of type B dissection and 2 instances of stent placement within the patient population.20
In a recent prospective, nonrandomized multicenter trial from the Disrupt PAD III observational registry, the safety and effectiveness of IVL therapy using the S4 catheter (Shockwave Medical) were assessed.21 The study authors reported an average acute gain of 2.0 ± 0.7 mm and a mean residual stenosis of 23.3% ± 12.5% at the 6-month follow-up.21 Furthermore, the Disrupt BKT II trial, involving 250 patients with critical limb ischemia and long calcified lesions from 40 global sites, is expected to publish its results later this year, further demonstrating the safety and effectiveness of IVL therapy in BTK PAD.
IVL in Iliac and Iliofemoral Artery Disease
Limited evidence exists regarding the utility of IVL in treating iliac and iliofemoral disease. A recent nonrandomized prospective study examined the feasibility and safety of IVL for iliac disease, utilizing a subgroup of patients extracted from the Disrupt PAD III observational registry.22 Out of the 118 patients included (with 200 moderate or severely calcified lesions ≥20 mm in length), 101 patients underwent treatment for CLI or claudication.22 The study concluded that IVL is a safe and effective option for calcified, stenotic iliac disease, with a final mean residual stenosis of 12.0% ± 12.1%.22 To support the efficacy of IVL in iliac and iliofemoral artery disease, Radaideh et al conducted a case series at a single center to evaluate its effectiveness.23 Despite encouraging results, the use of IVL for iliac and iliofemoral arteries prior to stenting is a novel approach, and experts worldwide are currently investigating the feasibility of this technique.
IVL in Common Femoral Artery Disease
The investigation into the utility of IVL for common femoral artery stenosis in patients with moderate-to-severe calcifications was initiated by Brodmann et al.24 The authors conducted a prospective multicenter study with core lab adjudication, focusing on severely calcified lesions to highlight the low residual stenosis and substantial acute luminal gain of 3.1 ± 1.3 mm achieved with IVL therapy.24 Among the 21 patients included in the study, 20% experienced minor (Type B) dissections that did not require additional treatment.24 This study’s findings were supported by a retrospective analysis conducted by Adams et al, which involved patients from 2 other centers.19
Meta-analysis
The evidence supporting the use of IVL therapy for extremity PAD is steadily increasing. Wong et al recently conducted a systematic review utilizing 9 studies to assess the efficacy and safety of IVL in severely calcified lower extremity PAD.25 The review revealed a significant reduction in vessel diameters between the pre-IVL and post-IVL stages (59.3% [95% CI; 53.3%-65.3%]).25 Severe vascular complications, such as flow-limiting or type D/E/F dissections, occurred in only 1.25% of patients.25 While current evidence is limited and studies focusing on upper extremity PAD are lacking, experts from around the world have reported encouraging outcomes with the use of IVL for PAD treatment.
Current Status of Technology and Future Use
IVL offers a groundbreaking option for treating calcified, stenotic lesions in patients with PAD, although its evaluation in a clinical setting is still incomplete. Currently, IVL has demonstrated efficacy in various vascular beds, challenging lesion subtypes, and complex patient populations, while maintaining an acceptable safety profile. However, most published studies on the safety of IVL in PAD have been limited by small patient cohorts. Despite promising results, most studies published on the safety of IVL in patients with PAD have had limited patient cohorts. While many predict that physicians will prefer the use of IVL for fracturing calcified plaques due to its ease of use, the clinical efficacy of IVL relative to established endovascular interventions, including atherectomy devices, as evaluated in level I clinical trials, and its cost-effectiveness are yet to be determined. Despite the ease of use of IVL for fracturing calcified plaques, the clinical effectiveness of IVL compared with established endovascular interventions, including atherectomy devices, as well as its cost-effectiveness, remain to be determined.12 Future clinical studies comparing IVL with non-IVL strategies using real-world registries will add more insight into the efficacy and safety of IVL and further establish its potential role in optimal treatment. If fully implemented into clinical practice, comprehensive training and education of cardiologists and interventional radiologists is necessary for effective use in patients.
Conclusion
IVL is a promising and innovative approach for treating calcification in peripheral arterial vessels. The existing studies provide strong evidence supporting the safety and efficacy of IVL. Improved luminal gain, vessel diameter, and mean residual stenosis, in addition to improvement in clinical indices of patients, has been observed in several studies.15-25 However, the current literature is limited in terms of sample size and exhibits significant clinical heterogeneity, particularly in the classification of calcified target lesions. Future studies should be conducted to comprehensively evaluate the efficacy of IVL in real-world settings, directly comparing it with atherectomy and other endovascular therapies. Additionally, longer-term follow-up is necessary to assess the sustained prognosis of patients.
Disclosures
From the 1Department of Medicine, University of Kentucky, Bowling Green, Kentucky; 2Department of Anesthesia, Beth Israel Deaconess Medical Center, Harvard Medical School, Boston, Massachusetts; 3Department of Medicine, CMH Lahore Medical College and Institute of Dentistry, Lahore, Pakistan; 4Department of Cardiology, University of Kentucky, Bowling Green Kentucky; 5Unity White County Medical Center, Searcy, Arkansas; 6Department of Medicine, Division of Cardiology, National Jewish Health, Denver, Colorado; 7Department of Interventional Radiology, University of Miami Health System, UM Miller School of Medicine, Miami, Florida; 8The NCH Heart Institute, NCH Healthcare System, Naples Florida; 9CHI St. Vincent Heart Institute, Little Rock, Arkansas.
The authors have completed and returned the ICMJE Form for Disclosure of Potential Conflicts of Interest. The authors report no conflicts of interest regarding the content herein.
Manuscript accepted January 19, 2024.
Address for Correspondence: Avnish Tripathi MD, PhD, 5315 W. 12th St., Little Rock, AR 72204. Email: avnish.tripathi@heartclinicarkansas.org