Noninvasive Evaluation of Patients With Chronic Limb-Threatening Ischemia
On behalf of the CLI Global Society Revascularization Committee
Pradeep K. Nair, MD1; Bret N. Wiechmann, MD2; Robert W. Tahara, MD3; Larry Diaz-Sandoval, MD4; Grigorios Korosoglou, MD5; Eric J. Dippel, MD6; Zola N’Dandu, MD7; Imraan Ansaarie, MD8; Fadi A. Saab, MD9; Erwin Blessing, MD10; Mazin Foteh, MD11; Mario D’Oria, MD12; Sreekumar Madassery, MD13; Anahita Dua, MD14; Mahmoud Abdelghany, MD15; John G. Winscott, MD16; David Yeager, DPM17; Tatsuya Nakama, MD18
ISSN: 2694-3026
J CRIT LIMB ISCHEM 2023:3(4):E127-E139. doi: 10.25270/jcli/CLIG23-00026
Abstract
Peripheral artery disease (PAD) of the lower extremities is a progressive atherosclerotic process that afflicts over 200 million people worldwide. Major amputation rates among those with chronic limb-threatening ischemia (CLTI) remain unacceptably high. Among those with occlusive lower-extremity PAD, a small proportion develop the most severe clinical sequelae of CLTI, which is associated with increased morbidity and higher mortality rates than patients with symptomatic coronary artery disease. Therefore, accurate, prompt diagnosis and treatment of CLTI is vital to improve prognosis and achieve limb salvage. This review focuses on specific noninvasive diagnostic tools that aid in corroborating findings on history and physical examination and provide guidance for the optimal CLTI management strategy.
J CRIT LIMB ISCHEM 2023:3(4):E127-E139. doi: 10.25270/jcli/CLIG23-00026
Key words: peripheral artery disease, chronic limb-threatening ischemia
Peripheral artery disease (PAD) of the lower extremities is a progressive atherosclerotic process that afflicts over 200 million people worldwide. Among those with occlusive lower-extremity PAD, 1% to 2% percent develop the most severe clinical sequelae of chronic limb-threatening ischemia (CLTI).1 This is defined by the presence of PAD in combination with rest pain, gangrene, or a lower limb ulceration of more than 2 weeks’ duration.2 CLTI is associated with increased morbidity and higher mortality rates than patients with symptomatic coronary artery disease.3
Nearly 25% of patients presenting with CLTI will suffer cardiovascular death within 1 year after initial diagnosis.4,5 Major amputation rates among those with CLTI remain unacceptably high at roughly 25%. Long-term survival after a major amputation is poor, with nearly 50% overall mortality at 1 year.5 Therefore, accurate, prompt diagnosis and treatment of CLTI is vital to improve prognosis and achieve limb salvage. After the history and physical examination, noninvasive diagnostic evaluation of CLTI is crucial to help direct the appropriate treatment algorithm. Demonstrating hypoperfusion via noninvasive means is recommended after a clinical suspicion for CLTI has been established. This can help avoid unnecessary invasive procedures in patients with adequate blood flow and symptoms related to other causes such as venous, neuropathic, infectious, hematologic, or pressure ulcers.
Imaging serves as an important and often necessary adjunctive tool to physiologic and perfusion assessment to help guide interventional therapies and identify targets for revascularization. While noninvasive diagnostic tools provide valuable information to help guide revascularization and surveillance, no single noninvasive test has been identified as the most important predictor of wound healing or major amputation for CLTI. Most operators utilize the modality with which their vascular laboratory has the most expertise. Given the anatomical complexities involved in CLTI endovascular intervention, while physiologic testing provides value, most operators will incorporate imaging to assist in formulating a revascularization approach. The remainder of this section will focus on specific noninvasive diagnostic tools that will aid in corroborating findings on history and physical examination and provide guidance for the optimal CLTI management strategy.
Physiologic Assessment
Ankle-Brachial Index
The ankle-brachial index (ABI) was first described by Yao in 1969.6 It is a simple, reliable, and widely available test to determine the severity of PAD. It is a ratio of the highest systolic pressure at the dorsalis pedis or posterior tibial artery (PTA; less commonly, the distal peroneal branch) divided by the highest systolic pressure of either the right or left brachial artery (Figure 1). Ankle pressures (APs) of less than 50 mm Hg are typical in CLTI. The ABI not only indicates the presence of PAD but is a marker for diffuse atherosclerosis, cardiovascular risk, and survival.7 In the supine and rested position, the ankle and brachial pressures should be equal, with the ABI equal to 1.00, but considering measurement variability, a lower limit of 0.95 is often accepted as normal. Many patients with CLTI have an ABI of 0.4 or less. However, falsely elevated ABIs are common among patients with diabetes mellitus or chronic kidney disease because of noncompressible crural arteries.8,9 An ABI over 1.4 should be reported as noncompressible, considered nondiagnostic, and warrant alternative testing (Table 1). Of particular importance are false elevations in ABIs between 0.4 and 1.4 in suspected patients with CLTI given the high concomitant prevalence of these comorbid conditions rendering noncompressible arteries. Yet, virtually all clinical trials of CLTI populations incorporate ABIs as a surrogate marker for clinical outcomes. Falsely elevated ABI values have been reported to be an independent predictor of major amputations.10 In this scenario, exercise ABIs can often help unmask a critical stenosis. Treadmill exercise can be attempted at 1 mph for 3 minutes with an ABI measured immediately afterward. For obvious reasons, however, it can be challenging to exercise a patient with PAD (and specifically CLTI) on a treadmill. In this scenario, leg/foot pumps or toe lifts are sometimes sufficient to unmask an otherwise innocuous resting ABI. A positive test is represented by a 20% drop in ABI or decrease in AP by more than 30 mm Hg from rest to exercise.6-9
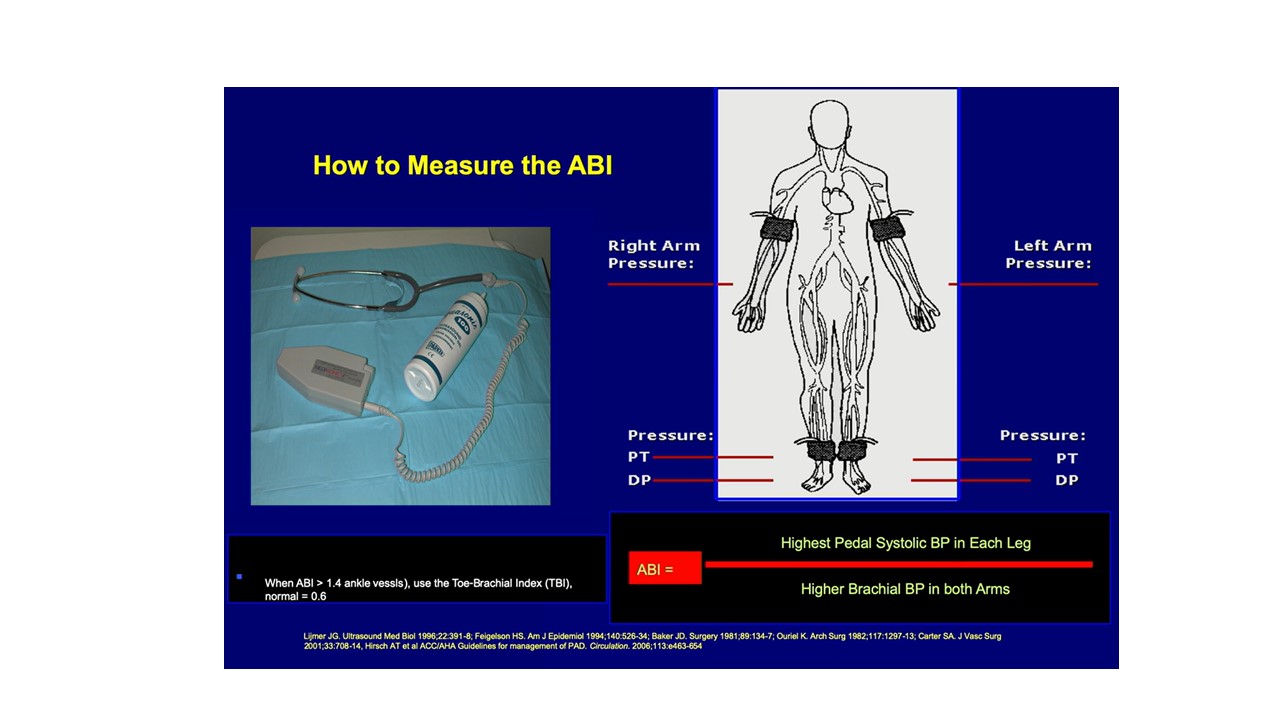
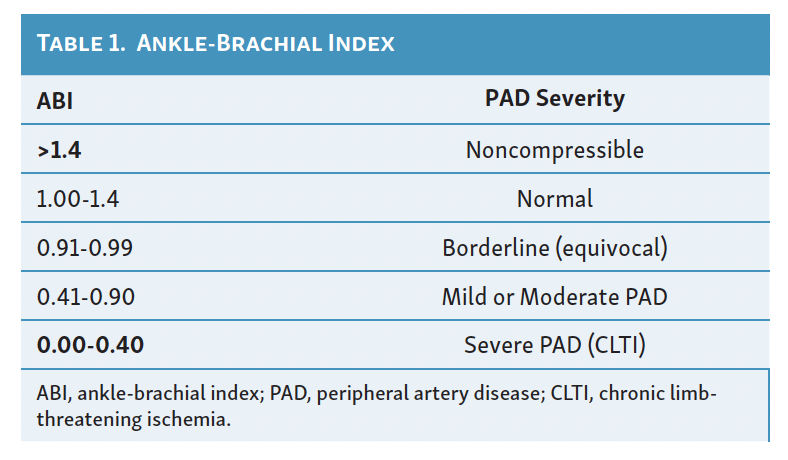
Segmental Limb Pressures
Localization of lesions to specific arterial segments (e.g. aortoiliac, femoropopliteal, infrapopliteal) can often be accomplished with segmental lower extremity blood pressures and Doppler or pulse volume recordings (PVRs).11,12 In some labs, this is typically performed at the time of ABI assessment. Typical segmental limb pressure measurements employ Doppler probes to measure the pulse volume waveform at segmental locations in the leg arteries. A change in the Doppler waveform from triphasic to biphasic to monophasic can help identify sites of arterial occlusive disease.13
To perform segmental limb pressures, a patient is rested for at least 15 minutes and placed in a supine position. Three-cuff or 4-cuff techniques have typically been employed for segmental pressure assessment. A 3-cuff technique uses 1 cuff above the knee, 1 below the knee, and 1 at the ankle. A 4-cuff technique uses 2 narrower cuffs above the knee to help differentiate aortoiliac and superficial femoral artery occlusive disease. The pedal vessel with the highest systolic pressure (dorsalis pedis or posterior tibial) is used. The minimal pressure that occludes the pedal signal for each cuff is measured. Index values for each level are obtained by the higher systolic arm pressure divided by the pressure obtained at each level. A 20 mm Hg or higher reduction in pressure is indicative of a flow-limiting stenosis when present between segments along the same leg or within segments at the same level in opposite legs. Segmental reductions by 20 mm Hg or higher in 1 limb is indicative of multilevel obstructive disease. Serial assessments that demonstrate an index change of more than 0.15 are generally indicative of disease progression. With the 4-cuff technique, a reduced high thigh pressure compared with the brachial pressure indicates proximal disease above the common femoral artery (CFA). Superficial femoral artery disease is noted when high thigh pressure is normal and low thigh pressure is reduced. This technique has been shown to correctly identify the level of the occlusive disease in 78% of limbs.13
There are some limitations with this method. Segmental limb pressures and PVRs cannot discern between stenoses or occlusions, as it may underestimate the extent of downstream lesions.14 The presence of collaterals utilizing this approach can obscure hemodynamically significant lesions. Hypertensive patients may accentuate the pressure gradient or decrease among those with a reduced cardiac output. As with ABI, cuff pressure can be artificially increased or uninterpretable by noncompressible vessels.
Pulse Volume Recordings
PVRs can be considered in patients with falsely high or uninterpretable cuff pressures due to vascular calcification. This modality measures changes in the plethysmographic waveform and can predict severity of limb ischemia. Cuffs are inflated to a constant, standard pressure, and volume changes in the limb segment beneath the cuff are reflected as changes in pressure within the cuff. This is converted to an electrical signal to produce an analog pressure pulse contour or PVR. Normal PVR waveforms demonstrate a sharp systolic upstroke and downstroke with a dicrotic notch. Alterations indicate upstream stenosis with mild disease demonstrating loss of the dicrotic notch and flatter slope and bowing of the downstroke. Blunted waveforms indicate severe upstream disease (Figures 2A and 2B). PVR amplitudes are affected by cardiac output and vascular tone, which limit interpretation within limbs of the same patients. Therefore, this test lacks reference values of normal to abnormal between different patients.
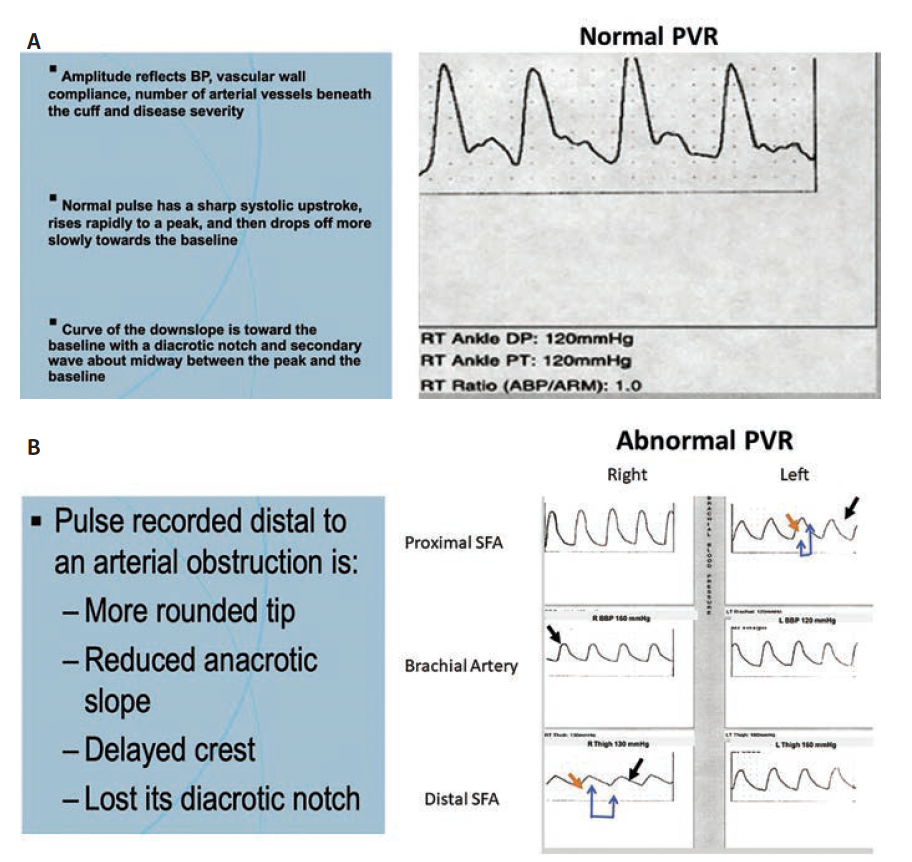
Toe Pressures
The measurement of toe pressures (TPs) is not routinely used in establishing a diagnosis of PAD, but it can be a valuable tool in the CLTI population to assess distal circulation. This evaluation is typically not affected by vessel calcification and can be a useful adjunct in those with an ABI over 1.4. There is a pressure gradient of 20 to 30 mm Hg that generally exists between the ankle and the toe. A TP is assessed by placement of a mini pneumatic cuff attached to a standard manometer around the base of the hallux. A continuous-wave Doppler flow detector or photoplethysmography is then used to determine when flow returns during cuff deflation. Studies indicate that TPs are more sensitive than APs in the diagnosis of CLTI and more predictive of amputation risk than APs.16,17 Typical for CLTI populations are TPs less than 30 mm Hg and almost never exceeding 40 mm Hg. Healing of toe ulcers and amputation sites is adversely affected when TPs are less than 30 mm Hg. If APs are unobtainable, it is customary to attempt a toe-brachial index (TBI). A normal TBI is 0.7 to 0.8, with less than 0.7 indicative of adverse limb events.17 APs and TPs are important components of validated classifications (WIfI: wound, ischemia, and foot infection), which has been shown to predict wound healing in CLTI.18
Limitations
The modalities mentioned above have limitations, especially within CLTI populations where extensive infrapopliteal disease and noncompressible vessels are present in high proportions. While ABI remains a first-line test in suspected patients with CLTI, it still has not been shown to be an accurate predictor of wound healing or major adverse limb events. In roughly 30% of patients with angiographically confirmed CLTI, the ABI is normal or noncompressible.19,20 Furthermore, APs appear to be poorly correlated with TPs. From a CLTI population of 237 patients with abnormal TPs, only 58% had abnormal ABIs.20 While TBI appears to be a better predictor of limb events than ABI in CLTI, strong evidence with core-lab adjudicated wound healing data remains absent. An absolute increase in TBI of 0.21 or more after endovascular revascularization has been shown to be associated with wound healing and reduced major limb events.21 However, the diagnostic accuracy of TPs across studies remains variable.22 Ulcerations or prior forefoot amputations clearly limit the utility of ABI and TBI assessment. Alternate modalities to assess perfusion can be sought in these instances. While ABI and TBI fail to localize arterial disease, other perfusion tools may aid in angiosome-guided revascularization.23
Perfusion-Based Assessments
After initial pressure evaluation or futility with noncompressible vessels, a vascular imaging modality is often performed to guide revascularization efforts in CLTI. Nevertheless, while these imaging tools can quantify stenosis severity at a particular anatomical location, they are limited in their ability to quantify perfusion to specific areas of the foot. Collateralization and anatomic variations can confound the ability to address perfusion beyond areas of stenosis. Additionally, distal arterioles, capillary networks, and microvascular disease are often contributing factors to wound healing that are unlikely to be addressed with traditional imaging techniques. Perfusion-based diagnostics are considered for the assessment of patients with CLTI when APs, TPs, and waveforms cannot be assessed. Perfusion-based assessment is an area of active investigation where many promising diagnostic tools are in development.24 Many of these modalities can assist in an angiosome-guided revascularization. Multiple studies support angiosome-guided revascularization being superior for wound healing and limb salvage rates compared with indirect revascularization, especially in the absence of collateral vessels.25 However, these tools can be influenced by a variety of confounding factors, and some remain experimental without extensive validation. Additionally, the availability of these tools remains limited. As a result, these modalities are not currently in routine use in most vascular laboratories.
Transcutaneous Oximetry
A commonly employed noninvasive perfusion tool in some vascular laboratories, transcutaneous oximetry (TcPO2) measures the partial pressure of oxygen at the skin surface. TcPO2 is measured by placing platinum oxygen electrodes on the chest wall and extremities and on any digit or amputation site being evaluated. Skin heating to 40 ˚C or more is required. Most devices use a temperature between 43 and 45 ˚C, which measures skin perfusion at maximal hyperemia. Oxygen molecules that diffuse through the skin to the surface are reduced between the electrodes of the probe to which voltage is applied. The reduction current produced is proportional to the reduced oxygen molecules, allowing partial pressure of oxygen to be assessed. The absolute value of the oxygen tension at the foot or a ratio of value of the foot to chest wall value can be used. A normal value at the foot is 60 mm Hg with a normal chest/foot ratio of 0.9.26 While the exact TcPO2 that predicts wound healing remains controversial, it is generally accepted that in the absence of edema and diabetes, oxygen tension over 40 mm Hg allows wounds healing. Values under 20 mm Hg are indicative of severe ischemia and require revascularization for wound healing. Values between 20 and 30 mm Hg remain at increased risk of chronic wound healing complications.27 The accuracy of this test is significantly limited by edema, skin and room temperature, sympathetic vasoconstriction, inflammation, and pharmacologic agents.28
Skin Perfusion Pressure
Skin perfusion pressure (SPP) is another noninvasive method of evaluating blood to unhealthy, below-the-ankle tissue in CLTI.29 SPP is most commonly measured by laser Doppler, radioisotope clearance, and photoplethysmography. This allows assessment of the microcirculation in the limbs at approximately 1.5 mm to 2.0 mm beneath the skin.30 The technique involves placement of a cuff that is inflated at the site of measurement. The pressure is slowly released and the movement of red blood cells, washout of isotope, or reappearance of pulsatile flow is recorded as the SPP..30,31 This method is unaffected by arterial calcification. Laser Doppler is the most commonly employed means of obtaining SPP given its fast, simple, and efficient method. Photoplethysmography is technically more challenging as deflection points in waveforms may be difficult to ascertain. Consensus regarding the threshold for the cutoff SPP is lacking. It appears that improved sensitivity as a predictor for wound healing occurs when SPP less than 30 mm Hg is utilized. This modality does not have strong evidence supporting its role as a diagnostic tool preintervention but has been shown to be useful in predicting wound healing after endovascular treatment.32
SPP has several limitations. Its reproducibility is poor as it is influenced by skin and body temperature, sympathetic tone, edema, and obesity. It also can be affected by motion artifacts, bony prominences, and veins.
Near Infrared Technology
Near infrared (NIR) spectroscopy is a noncontact and noninvasive technique that can provide regional tissue oxygen content or delivery (Figure 3). This technique spectrally analyzes changes in the relative concentrations of oxyhemoglobin and deoxyhemoglobin within tissues. NIR spectroscopy can estimate the percent oxygen saturation of hemoglobin in nonpulsatile vessels (veins and capillaries) within tissue at various depths, including the muscular layer. Its ability to predict wound healing represents an area of investigation and intrigue.
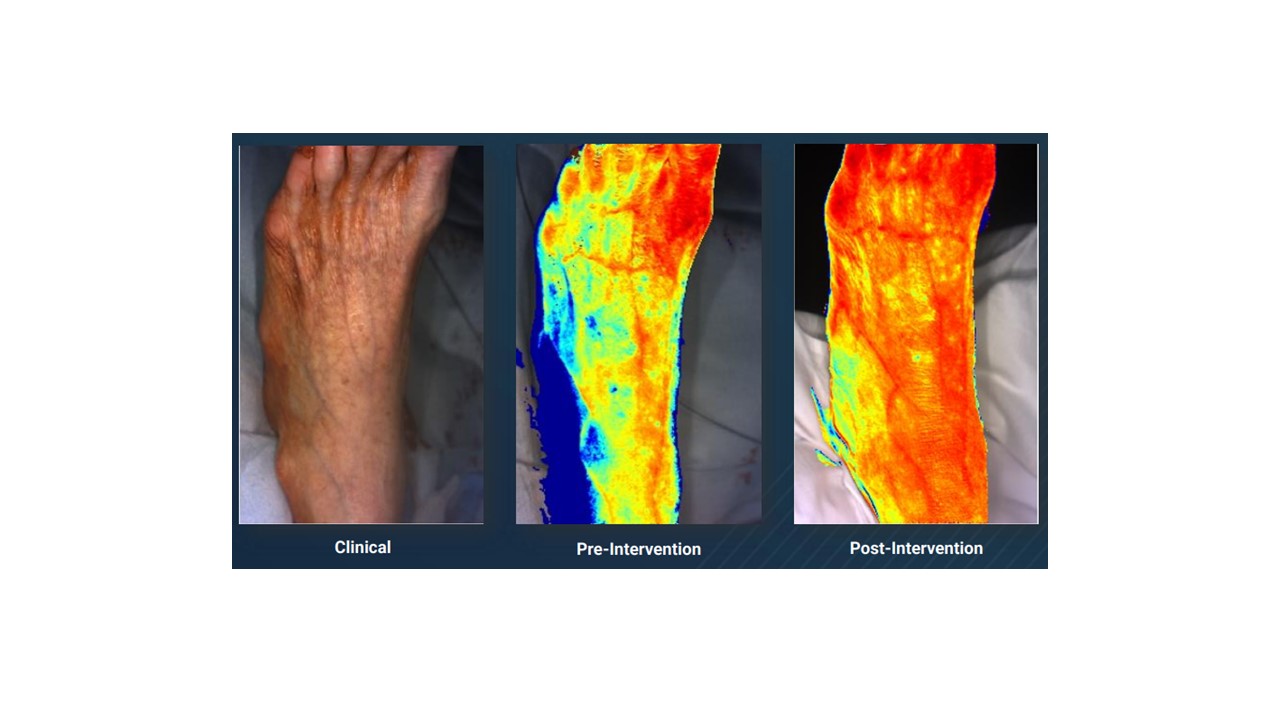
NIR fluorescence has been used in conjunction with indocyanine green (ICG) to assess tissue blood flow. This imaging technique measures fluorescence in the NIR light spectrum (700-1000 nm), characterized by low tissue autofluorescence and deep tissue penetration.33 ICG has a peak emission of 814 nm and remains confined in the intravascular compartment due to its ability to bind to plasma proteins. After ICG has been intravenously administered, the camera measures NIR fluorescence intensity over time. These techniques are limited by availability and limited clinical data in CLTI populations. The use of ICG also has limitations among those with iodine and sulfa allergies.
Perfusion With Noninvasive Imaging
Duplex ultrasound (DUS), computed tomography (CT), and magnetic resonance (MR) can effectively assess blood flow throughout the lower extremity arterial system. However, assessing vessel patency and flow is only a surrogate marker for perfusion. Methods of perfusion evaluation utilizing CT and MR are currently considered experimental. Perfusion CT is widely used in cerebral imaging to aid in the implementation of thrombolytic therapy, tumor characterization, and evaluation after subarachnoid hemorrhage.34 Likewise, arterial spin labeling MR has been used for 3 decades to measure cerebral blood flow. Arterial spin labeling institutes a 190-degree radiofrequency inversion pulse that leads to inversion of the net magnetization of water, which creates a reduced signal intensity referred to as the tagged image. Images with and without a tagged image are obtained, creating the basis for perfusion assessment. Another MR assessment of perfusion incorporates the blood-oxygen level-dependent (BOLD) technique.35 Cerebral and cardiac perfusion have been assessed for many years using BOLD imaging, where the MR can delineate the magnetic properties of oxygenated and deoxygenated hemoglobin. The benefit of the MR technique over CT is the lack of radiation or iodinated contrast exposure.36 Additionally, using arterial spin labeling or BOLD techniques does not require gadolinium-based contrast agents. The ability to translate these modalities for patients with CLTI may assist in limb salvage efforts and wound healing.
Noninvasive Vascular Imaging
Duplex Ultrasound
Duplex ultrasound is a primary bedside noninvasive tool to document and describe arterial anatomy and evaluate the severity of existing PAD. It is commonly used as a first-line imaging modality in the evaluation of patients with CLTI, providing both anatomic and physiologic information, and has undergone a long progressive development since its first use in the 1950s. Doppler ultrasound has evolved from pure flow sensing to Doppler waveform analysis, and past the initial attempts at tissue and blood flow imaging to a modality that now gives accurate and detailed imaging and waveform analysis.38 Duplex imaging was first used in 1974 and developed as a way to fuse the anatomic B mode grayscale imaging with color flow Doppler imaging.39 Duplex may be used to different degrees depending on the practice and the operator. It may serve as a preliminary assessment before proceeding directly to a more complex modality, such as CT angiography (CTA)/MR angiography (MRA), or a more detailed examination and evaluation, which may or may not require additional imaging, or in some centers even as the exclusive preoperative diagnostic and operative planning imaging modality.40
Logistically, the performance of high-quality duplex exams for CLTI requires appropriate instrumentation (ultrasound machine); competent, trained, and experienced technologists (registered vascular technologists [RVTs]) or physicians; a room or facility with adequate space and supplies; and an interpreting physician with appropriate training and experience. Ultrasound machines are commonly available in many configurations from a multitude of vendors and various price ranges. These configurations include both hardware and software options that give users a variety of choices to best suit the type and depth of exams being conducted.
Hardware considerations include the footprint of the unit and range from large and barely mobile machines to laptop or tablet-based units. While an exhaustive discussion of the variations and options for ultrasound machines is beyond the scope of this publication, a high-quality, full-size diagnostic unit (as opposed to a laptop or tablet-based unit), equipped with at least 7 to 9 MHz and 11/12 MHz or 15 MHz linear transducers, and installed with a full lower extremity arterial software package, is recommended to conduct a proper CLTI exam, mapping, and for both preoperative and intraoperative imaging.41,42,43
There are multiple routes for training and certification of vascular ultrasound technologists, but satisfactory completion of a vascular-specific credential such as the ARDMS RVT should be considered a basic requirement for a competent technologist performing duplex exams of this type. The RVT should regularly perform exams on patients with CLTI and have regular feedback and continuing medical education in accordance with published societal recommendations.44,45
Physically, the room space in which these exams are conducted should be large enough to comfortably accommodate the patient in a supine position, the technologist, and the equipment. Adequate draping, linens, and a warm, quiet environment are needed to assure an adequate and detailed examination.46,47
Finally, the physician interpreting the exam must have training and experience as obtained in accredited residency/fellowship training in a major applicable specialty such as vascular surgery, radiology, or interventional cardiology. The ARDMS Registered Physician in Vascular Interpretation credential can serve to verify that the interpreting physician has both been trained and has reached a basic level of competence in interpreting these exams.48
Exam Conduct
Multiple recommendations for the appropriate performance of a lower extremity diagnostic duplex exam are well documented in the literature and by cognizant specialty societies such as the Society for Vascular Ultrasound (SVU). Appropriate guidelines and protocols are available from SVU and other specialty and accreditation organizations.49 Duplex examination of patients with CLTI should assess and document the status of inflow into the affected leg; include detailed descriptions documenting waveforms, color flow, velocities, and grayscale images in the infrainguinal segment both above and below the knee; and optimally, describe and document an assessment of inframalleolar outflow and anatomy to include named pedal arteries and anterior and posterior outflow vessels.
Inflow Assessment
Evaluation of the distal external iliac artery and CFA using color flow and waveforms should give an accurate evaluation of inflow status. Triphasic waveforms with no evidence of post-stenotic turbulence and minimal evidence of plaque on grayscale should be reassuring of adequate inflow. Conversely, monophasic weak flow or obvious stenosis/post-stenotic turbulence and/or grayscale-apparent plaque burden should prompt a more detailed proximal evaluation (Figure 4). Body habitus or bowel gas may render difficulty in assessing the proximal vessels. When this occurs, CTA or MRA may be necessary for a complete anatomic evaluation or to plan inflow revascularization.50
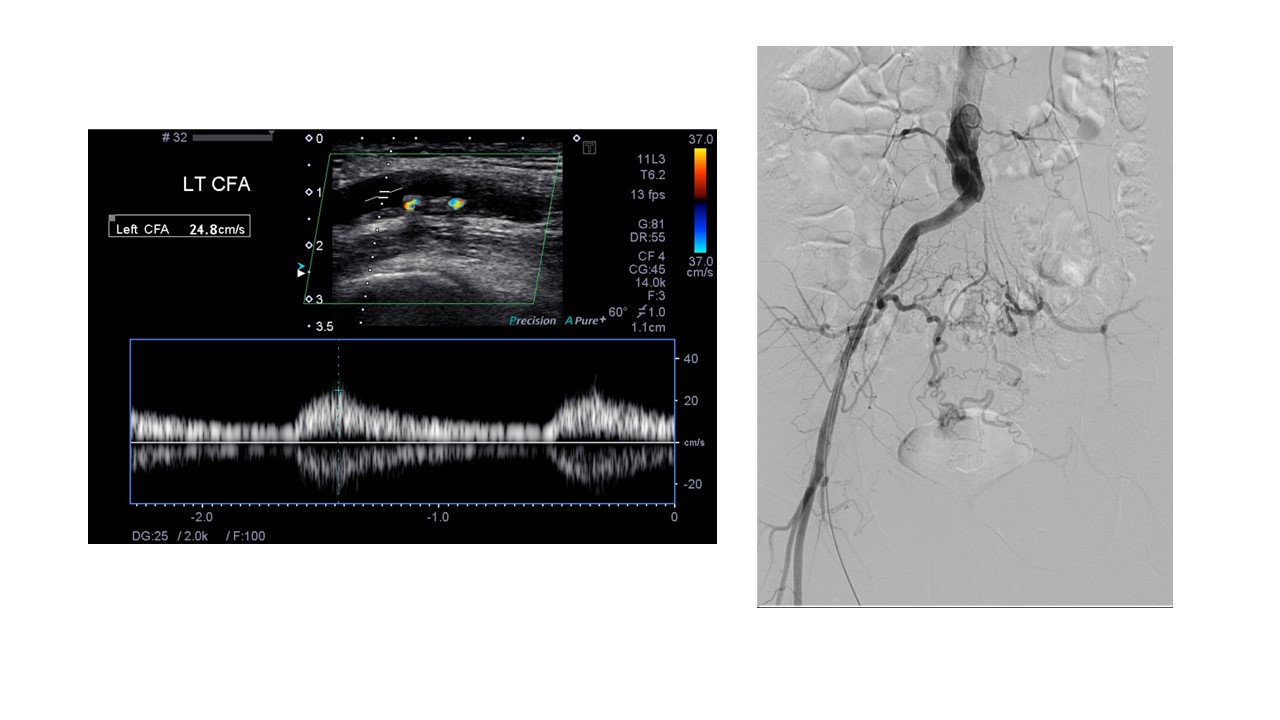
Infra-inguinal Above-Knee/Below-Knee Evaluation
Standard protocols for the evaluation of the femoral arteries (CFA, profunda femoris artery, superficial femoral artery), popliteal arteries, and named tibial arteries are in wide usage and are noted above. Documentation of waveforms, color flow, complete occlusions, stenoses, aneurysms, and collaterals are all critical to developing a complete picture of the disease burden and an understanding of potential treatment options for CLTI. Weak, low-velocity, monophasic waveforms are generally consistent with a CLTI diagnosis, and in most cases multiple-level stenoses or occlusions will be present.37,47 A detailed description and images showing the degree of medial arterial calcification, extravascular tissue calcification, and some assessment of plaque morphological characteristics should be documented. Plaque calcification and patterns of occlusion and stenosis when combined with degree of tissue loss may guide determination of the interventional approach or need for further noninvasive diagnostics. Assessment and documentation of potential access points are required both at the groin for antegrade/retrograde femoral access and/or at the ankle/foot for potential tibial/pedal access. If the study will be used as a primary intervention/operative planning study, the details of intraluminal plaque morphology, calcification, and thrombus will need to be thorough and complete to facilitate access point and device selection for endovascular therapy.37 This may also serve to stratify whether a primary endovascular approach is even feasible and/or desirable in the eyes of the intervening physician. One recommendation that may be useful is a hand-drawn map that can be used to assist in visualizing the severity and pattern of disease present for both planning and intraprocedural reference (Figure 5).
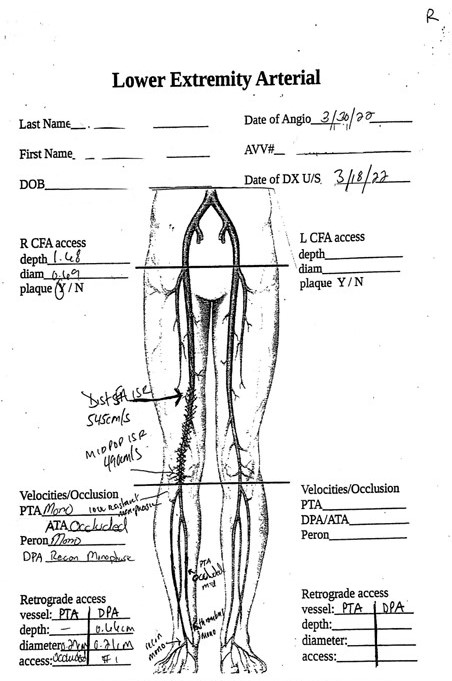
Inframalleolar Outflow
Evaluation and documentation of inframalleolar arteries are now recognized as being critical parts of evaluating and treating some patients with CLTI. Documentation of the anatomy, disease
burden, and flow pattern to affected areas of the foot may help the operator understand the available ways to approach revascularization and when angiosome-directed revascularization may be necessary.48,49 Patients with diabetes and patients with renal failure are typically more likely to have extensive disease in the foot and may require more detailed imaging and treatment for successful limb salvage.50 For a patient with great toe or heel gangrene, weak monophasic flow into the inframalleolar PTA and into the medial and lateral plantar arteries despite multiphasic flow into the dorsalis pedis artery implies poor or discontinuous/occluded pedal arch flow. This might prompt the operator to consider direct PTA-angiosome revascularization. Similarly, great toe gangrene in a patient with an obvious focal medial plantar artery occlusion might well prompt inframalleolar treatment/reconstruction, even with good flow to the ankle.
In addition to standard methods of assessing flow, plantar or pedal acceleration time (PAT) has recently been described and discussed as a method to both characterize disease severity/amputation risk and evaluate treatment adequacy.51 Briefly, the systolic acceleration time is calculated and assigned to 1 of 4 classes based on time. Class 4 (>225 msec) is the most severe, and improvement from Class 4 to Class 2 (121-180 msec) was associated with limb salvage in 81% of the study population. Conversely, the remaining 19% who failed to improve PAT ultimately underwent above-ankle amputation of the affected limb.52
Thorough assessment of inframalleolar arteries requires a highly experienced RVT, and the technical challenge of obtaining high-quality images and documenting pedal vessels, pedal arches, and describing flow patterns should not be underestimated—this is truly “varsity level” vascular imaging.
Limitations of Duplex Imaging for CLTI
Duplex evaluation of this complex group of patients is typically limited by either patient and patient tissue characteristics or the experience of the facility/technologist scanning the patient. Heavily calcified arteries can lead to so much signal dropout that waveforms and color are essentially unobtainable in the affected segment. Additionally, heavily calcified tissue, as frequently observed in the subgroup of patients with diabetes and/or renal failure, may severely limit the ability to visualize plaque and arterial wall characteristics. Deeper vessels, particularly in heavier patients, often magnifies calcification issues and makes imaging particularly challenging, even when using lower frequency, more penetrative transducers.
Most RVTs will have the requisite training, experience, and familiarity to adequately perform duplex imaging to assess inflow of the major named conduits above and below the knee. Experience in the distal tibials and inframalleolar vessels is not uniformly present outside of the minority of operators or facilities where inframalleolar intervention and/or bypass is regularly performed. This is likely the most significant and frequent technologist limitation in whether inframalleolar flow and anatomy can be adequately assessed and documented by duplex. In this situation, the physician may require other forms of imaging such as MRA or formal digital subtraction angiography (DSA) for both diagnosis and planning purposes.
In summary, duplex is a highly useful, safe, accurate modality for diagnosis and treatment of patients with complex CLTI. Equipment needs are limited and substantially less expensive than modalities such as CTA/MRA. No contrast or radiation is necessary, and duplex may serve as the sole diagnostic modality in facilities that have the requisite experience and expertise to complete imaging of the entire lower extremity, including inframalleolar vessels.
Computed Tomography Angiography
While DUS has some advantages in the assessment of anatomy in patients with CLTI, its limitations in the deeper iliac segment and in assessing the smaller, frequently calcified tibial vessels presents challenges. CTA tends to be less technologist-dependent than DUS, is less invasive than standard peripheral angiography (without the need for arterial puncture) and is less expensive. The role of CTA in the noninvasive evaluation of the patient with CLTI remains one of the most frequently used measures to assess arterial anatomy that covers the aortoiliac, femoropopliteal, and infrapopliteal vessels. CTA has the ability to assess intraluminal features of vascular structures but also provides extraluminal detail and evaluation of adjacent, potentially relevant anatomy. Previously limited longitudinal coverage of multiple arterial segments has been mitigated by the development of better scan technology. The evolution from early-generation, single-slice scanners and single-station axial imaging to current multidetector/dual-source scanners and spiral (helical) scan technique for rapid and dynamic scanning allows better source imaging. The speed of these scanners allows for single bolus intravenous iodinated contrast injection with a single breath-hold to cover the areas of interest. These technical advancements reduce the need for repeat injections from bolus timing error and artifacts from patient motion and misregistration. Improved software algorithms can create high-quality reformatted images to evaluate in multiple planes to simulate standard on-table angiographic views. Finally, although use of alternative imaging is regionally variable in the United States, the wide availability of CT technology in most communities offers the potential for diagnostic use in the CLTI population.53
The high spatial resolution of CTA allows for fairly detailed plaque assessment (primarily soft vs calcified plaque) as well as detection of intraluminal thrombus, dissection, and aneurysm formation. Imaging protocols for CTA in patients with CLTI will vary based on existing equipment, and specific details are beyond the scope of this article. Generally, newer scanners allow for slice thicknesses as low as 0.5 mm, although from a practical standpoint typical slice thickness is 1.25 mm to 2.5 mm for peripheral CTA. This balances scan speed and efficiency with
maintenance of longitudinal resolution and therefore source image quality. Luminal enhancement is an additional component of source image quality that is critical in the production of a diagnostic study. This is directly related to bolus volume and timing. Patient considerations including intravenous access site, intravenous gauge size (and therefore injection rate tolerance), and cardiac output may influence volume and timing. Software for identifying maximum lumen enhancement (“peak detection”) is employed on most CT systems to generate the highest possible contrast resolution. This summary of data acquisition is important to consider to appropriately plan intervention in the CLTI population.
Interpretation of the source images is complemented by review of the various multiplanar reformatted (MPR) images including curved MPR, 3D volume-rendered images, and maximum intensity projections (Figures 6A and 6B). These post-processing techniques can be extremely invaluable in evaluating details of vessels that may contain dense calcification or that may be oriented in a nonaxial plane. While the reconstruction of the source images may compromise certain features of CT imaging (eg, spatial resolution), incorporating these additional images into procedure planning is common and quite helpful.
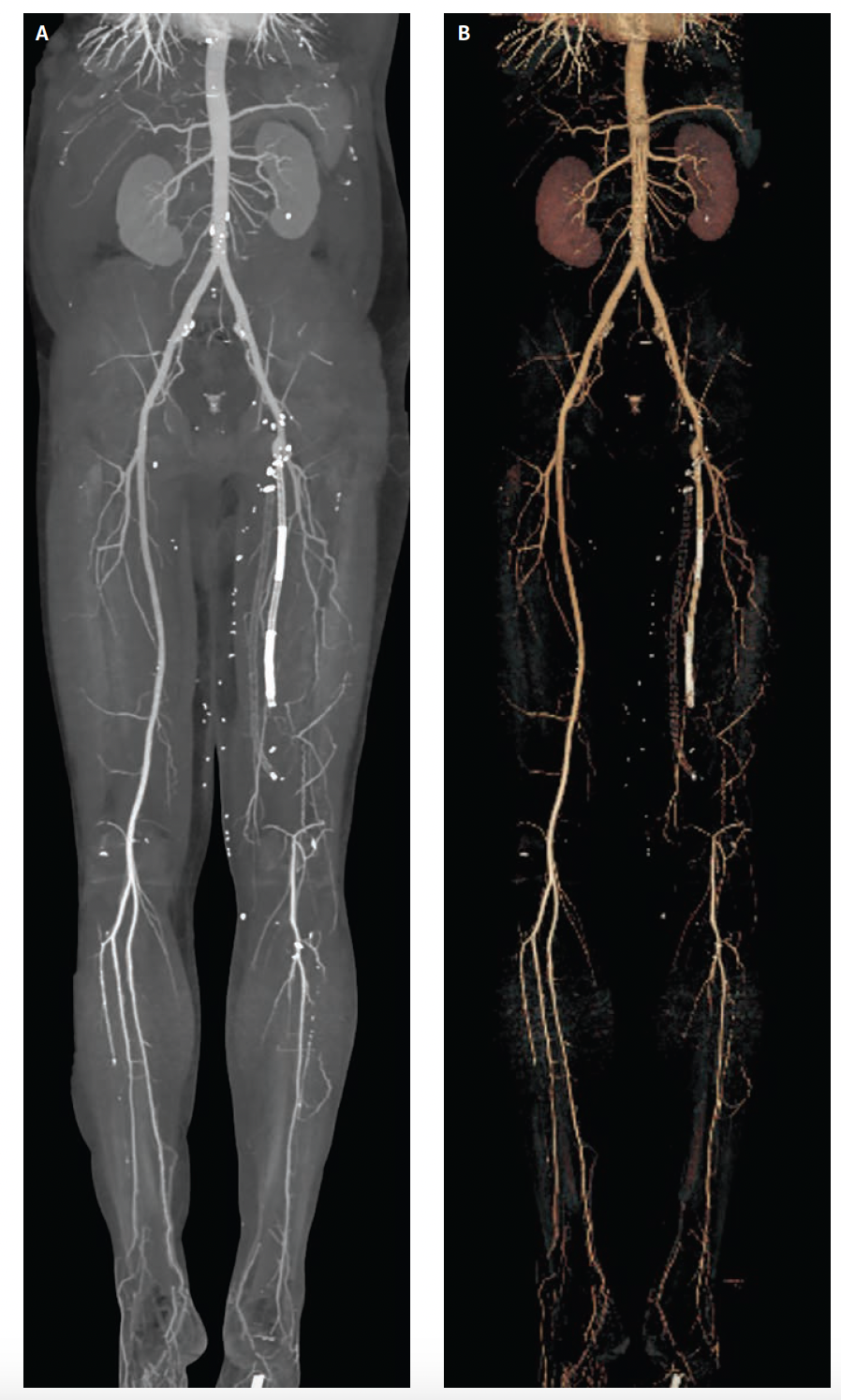
The limitations of CTA are well known and include the need for use of iodinated contrast, dense calcification that may compromise luminal evaluation, and the use of ionizing radiation. Chronic kidney disease (CKD) is highly prevalent in the CLTI population, and therefore contrast media exposure in the diagnosis and treatment of CLTI is not without risk and its use should be cautious. In fact, with use of alternative arterial contrast agents (carbon dioxide), the total iodinated contrast volume of lower extremity diagnostic angiography can frequently be lower than standard peripheral CTA (typically at least 100 cc iodinated contrast volume). As with any key decision in this challenging patient group, case selection and a careful risk vs benefit assessment is paramount to overall success. Dense vessel calcification can result in “blooming artifact,” which can result in overestimation of stenosis. This effect is somewhat magnified in the tibial vessels due to the higher percentage of calcium relative to native vessel size. Some of this may be mitigated by post-processing techniques or adjusting the viewing “window” and “level.”
Literature Assessment of CTA in PAD/CLTI
Clinically important findings from preprocedural CTA have been well documented. The diagnostic accuracy of CTA has been shown to be slightly higher in the larger, more proximal vessels. Al-Rudaini et al noted an overall accuracy of CT angiography in the femoropopliteal segments of 95.2% with an accuracy of 94.5% in the infrapopliteal vessels.54 In a study evaluating newer 256-slice CTA for detecting significant occlusive disease, Mishra et al noted a sensitivity of 91.6% of detecting infrapopliteal lesions, which was comparatively less than the aortic/femoral segment, especially in the presence of calcification.55
In a metanalysis of 673 patients in 12 CTA studies by Jens et al, CTA had a sensitivity and specificity for detecting hemodynamically significant, greater than 50% stenosis of 96% and 95%, respectively.56 No difference was noted between intermittent claudication and patients with CLTI. While diagnostic accuracy in terms of percentage stenosis in the tibial vessels is seemingly less, the ability of CTA to detect calcium in the tibial vessels can be used to help predict procedural success as well as major adverse limb events, unplanned amputation, and periprocedural mortality.57-59 In addition, the extent and severity of circumferential calcifications can be assessed more precisely by CTA compared with fluoroscopy or DSA.
Magnetic Resonance Angiography
The basic premise behind vascular MR imaging is based on intrinsic tissue parameters and their respective responses to the magnetic pulse sequences during image acquisition. In addition, MRA can use flow-enhanced signal variation to provide dynamic and directional information. Different imaging sequences and protocols can be without or without contrast media. Non-contrast enhanced sequences (NCE-MRA) rely on the flow of blood (eg, time of flight, phase contrast technique), whereas contrast-enhanced MRA relies on the usual identification of the contrast agent within the lumen of the vessel. NCE-MRA tends to provide excellent images with good signal for postprocessing and review, provided there is a relatively high flow state. One of the challenges with this technique in patients with PAD/CLTI is that in the presence of occlusive disease, flow patterns may indeed be sufficiently slow that there is significant signal dropout and therefore images may be nondiagnostic. In addition, given the need to scan over the length of the lower extremities and potential asymmetric flow, timing with NCE-MRA techniques may be difficult and result in long exam times. This technique is also subject to patient motion artifacts. As a result of these factors, CE-MRA has evolved into the technique of choice for patients requiring MRA before intervention.60 Most institutions use gadolinium-based agents for intravenous administration prior to CE-MRA. The volume of contrast agent is much less with CE-MRA (30 cc) than for typical CTA (100 cc-150 cc) and as with CTA, bolus timing (at “peak” enhancement) is critical for completing a fully diagnostic study. Venous contamination as a result of suboptimal scan time can obscure the intended imaging target, which is particularly notable in the tibial vessels. Additional strategies to mitigate this problem, such as the 3-station “bolus-chase” and “time-resolved” technique, can be employed to provide a temporal assessment of femoropopliteal and tibial artery flow, a key factor in patients with CLTI.61 Newer techniques are being evaluated to optimize arterial images, decrease venous signal, minimize motion artifact, and adjust for abnormal flow patterns (Figure 7).62,63
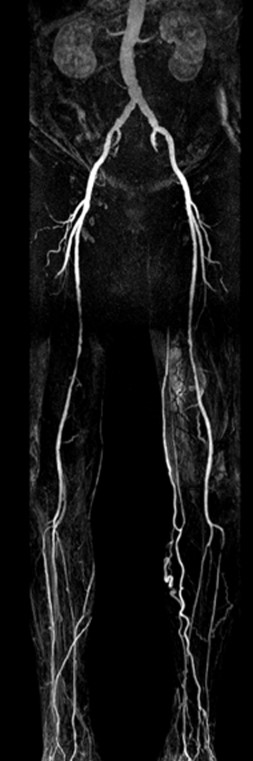
Advantages of MRA include the lack of ionizing radiation, which may be of concern in patients who may undergo multiple procedures requiring lengthy radiation-based interventions. Some studies suggest that MRA performs better than CTA regarding calcification, depending on the technique employed, but this remains an area of debate. The limitations of MRA in patients with CLTI include a similar concern in those CLTI patients with CKD. Reports of nephrogenic systemic fibrosis (NSF) have stoked concerns about using gadolinium contrast agents in this group, although skepticism over the true incidence of gadolinium-related NSF has grown recently.64 This has also led to the development and investigation of alternative MRA contrast agents.65 The overall spatial resolution of MRA is less than CTA, especially with NCE-MRA techniques. Additional limitations of MRA for peripheral vascular imaging include significant signal dropout and artifact related to metallic implants, in particular vascular stents that may indeed be in the target area. Finally, cardiac pacers and patients with claustrophobia may not be able to tolerate MRA.
Literature Assessment of MRA in PAD/CLTI
Collins et al showed that for the detection of stenoses over 50%, CE-MRA had high overall diagnostic accuracy and is more accurate for detecting stenoses/occlusions above the knee than the tibial and pedal vessels.66 In the Jens meta-analysis evaluating detection of hemodynamically significant disease from the aorta to the tibial arteries,56 CE-MRA showed a sensitivity of 93% and a specificity of 94%, with very few nondiagnostic studies (1%-2% all modalities), and excellent interobserver agreement.56
Conclusions
Noninvasive testing is a crucial step for the evaluation of patients with CLTI. Physiologic assessments such as ABI and TBI can be useful in establishing a diagnosis and need for revascularization, yet these tests serve primarily as adjuncts to imaging and remain a prominent metric for efficacy assessment in clinical trials. The CLTI population has unique comorbidities that can make physiologic assessment challenging to interpret. Noninvasive perfusion tools would be an ideal way to assess angiosomal distribution of perfusion and surveillance post revascularization. However, these tools are not yet widely available, and many remain experimental.
DUS has the advantage of being readily available and requires no radiation or administration of potentially nephrotoxic agents to the patient. Assessment of endoluminal anatomy is possible and can aid in formulating a revascularization strategy. Interpretation and acquisition require formal training. Reproducibility, reliability, and quality of scans unfortunately can be variable depending on the center and level of expertise. Furthermore, calcification can make imaging challenging from shadow artifacts, and ulcers can yield discomfort during scan acquisitions. Inflow vessels can also be difficult to adequately assess depending on body habitus. Nevertheless, DUS has clear benefits in evaluating patients with CLTI and in many cases is sufficient in planning revascularization.
CTA and MRA provide meaningful preprocedural information to help plan intervention in patients with ischemic rest pain and minor/major tissue loss. This includes determining the best revascularization strategy (surgical vs endovascular), choice of initial and additional access site (if endovascular),67 choice of bypass target (if surgical), and even predicting potential technical success, limb outcomes, and mortality. Both CTA and MRA offer advantages and disadvantages, and the choice of noninvasive arterial imaging, whether DUS, CTA, or MRA, is likely to be patient-, physician-, and center-specific. Considerations include patient comorbidities, presence of CKD, degree of calcification, previous interventions (stents, other metallic implants), and potential contrast reactions.
Ultimately, where noninvasive imaging fits in the overall assessment of the patient with CLTI will depend on availability and ability to acquire reproducible, high-quality diagnostic studies to improve the overall chance of limb salvage and reduce mortality related to CLTI.
Disclosures
From 1Cardiovascular Institute of the South, Houma, Louisiana; 2Vascular & Interventional Physicians, Gainesville, Florida; 3Allegheny Vein & Vascular, Bradford, Pennsylvania; 4Borgess Heart Institute, Kalamazoo, Michigan; 5GRN Hospital Weinheim, Weinheim, Germany; 6Vascular Institute of the Midwest, Davenport, Iowa; 7Oschner Health System, Kenner, Louisiana; 8Ansaarie Cardiac & Endovascular Center of Excellence, East Palatka, Florida; 9ACV Centers, Grand Rapids, Michigan; 10University Hospital Hamburg, Hamburg, Germany; 11Baylor Scott & White Heart Hospital, Plano, Texas; 12University Hospital of Trieste, Trieste, Italy; 13Rush University Medical Center, Chicago, Illinois; 14Massachusetts General, Boston, Massachusetts; 15Cleveland Clinic, Cleveland, Ohio; 16The University of Mississippi Medical Center, Jackson, Mississippi; 17Morrison Community Hospital, Morrison, Illinois; 18Tokyo Bay Medical Center, Tokyo, Japan
Disclosure: The authors have completed and returned the ICMJE Form for Disclosure of Potential Conflicts of Interest. The authors report no conflicts of interest regarding the content herein.
Manuscript accepted October 5, 2023.
Address for Correspondence: Pradeep Nair, MD, Cardiovascular Institute of the South, 225 Dunn St, Houma, LA 70360. Email: pradeep.nair@cardio.com